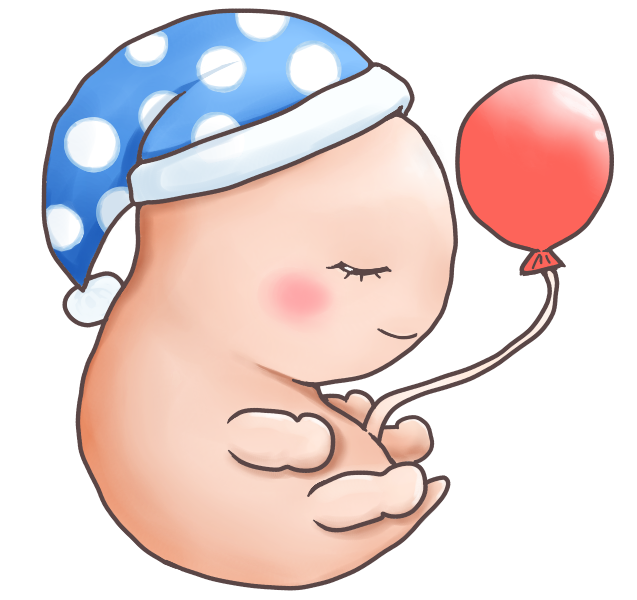
- 最新画像技術を用いて、ヒトがどのように形作られるかの ’見える化’に取り組んでいます…
- 研究する仲間(学生・大学院生・研究者)募集中です!
- 総合医療科学パンフレット(pdf)ができました
|
||||
![]()
![]() 第78回日本人類学会で発表しました。(2024.10.12-14, 大阪) ■ 倭 友希、松田 幸樹、松林 潤、金橋 徹、今井 宏彦、米山 明男、山田 重人、高桑 徹也 :ヒトの胚子期における足部・手部の形態形成 ■ 青江 春菜、金橋 徹、今井 宏彦、大谷 浩、山田 重人、高桑 徹也:ヒト胎児期初期における下顎・歯胚の形態形成の定量的解析 ■ 熊谷 美優、金橋 徹、今井 宏彦、大谷 浩、多賀 厳太郎、高桑 徹也:高解像度 MRI を用いたヒト胎児における大脳基底核原基の形成過程の検討 岩佐さんの僧帽筋形成についての論文がJ Anatomyに受諾されました。MRI_DTIを用いて僧帽筋形成過程を、外観だけでなく、椎体レベルごとの筋線維の走行まで観察しました。また、論文内の図が表紙に採用されました。
![]() 69. Iwasa Y, Kanahashi T, Imai H, Otani H, Yamada S, Takakuwa T. Human trapezius muscle development during early fetal period. J Anatomy 2024, 245, 663-673, doi: 10.1111/joa.14116 Abstract This study aimed to observe human trapezius muscle (TpzM) development during the early fetal period and apply diffusion tensor imaging (DTI) analysis to describe the muscle architecture that leads to physiological functions. Human embryonic and early fetal specimens were selected for this study. TpzM was first detected at Carnegie stage 20. The position of the TpzM changed with the formation of the scapula, clavicle, and vertebrae, which are its insertions and origins. DTI revealed the fiber orientation from each vertebral level to dissect each muscle. Fiber orientation in the ventral view gradually changed from the cervical to thoracic vertebrae, except for the middle part at which the insertions changed, which was almost similar in all early fetal specimens. The TpzM volume increased from C1 to C7 in the upper part, reached local maxima at C6 and C7 in the middle, and then decreased. These muscles can be categorized into three parts according to their insertions and presented with the features of each part. The fiber orientation and distribution of the three parts at the vertebral level were almost constant during the early fetal period. The border between the upper and middle parts was mainly located around the C6 and C7 vertebral levels, whereas the middle and lower parts were between the Th1 and Th2 vertebral levels. A three-dimensional change in the fiber orientation in the upper part of the TpzM according to the vertebral level was noticeable. Our data will help to elucidate the developmental processes of TpzM. ![]() 磯谷さんの修士論文がAnatomical Recordに掲載されました。 胎児循環に特有の胎盤から心臓にむかう静脈路(臍帯静脈、門脈洞、静脈管、下大静脈)について、領域による特徴を高解像度デジタルデータ(MRI, CT)から得られた立体再構成像と組織像を用いて検討しました。
67. Isotani N, Kanahashi T, Imai H, Yoneyama A, Yamada S, Takakuwa T. Regional differences in the umbilical vein and ductus venosus at different stages of normal human development. Anat Rec (Hoboken), 2024, 307, 3306-3326.DOI:10.1002/ar.25421 During the fetal period, oxygenated blood from the placenta flows through the umbilical vein (UV), portal sinus, ductus venosus (DV), and inferior vena cava (IVC) to the heart. This venous route varies regionally in many aspects. Herein, we sought to characterize the venous route’s morphological features and regional differences during embryonic and early-fetal periods. Twenty-nine specimens were selected for high-resolution digitized imaging; 18 embryos were chosen for histological analysis. The venous route showed a primitive, large, S-shaped curved morphology with regional narrowing and dilation at Carnegie stage (CS) 15. Regional differences in vessel-wall differentiation became apparent from approximately CS20. The vessel wall was poorly developed in most DV parts; local vessel-wall thickness at the inlet was first detected at CS20. The lumen of the venous route changed from a non-uniform shape to a relatively round and uniform morphology after CS21. During the early-fetal period, two large bends were observed around the passage of the umbilical ring and at the inlet of the liver. The length ratio of the extrahepatic UV to the total venous route increased. The sectional area gradually increased during embryonic development, whereas differences in sectional area between the DV, UV, and IVC became more pronounced in the early-fetal period. Furthermore, differences in the sectional area between the narrowest part of the DV and other hepatic veins and the transverse sinus became more pronounced. In summary, the present study described morphological, morphometric, and histological changes in the venous route throughout embryonic and early-fetal development, clarifying regional characteristics. 放射光位相CTシンポジウム日時:2024/9/9(月) 13:00-18:10 プログラム: ![]() 13:00-13:15 開会のご挨拶 ![]() オープンキャンパスが開催されました。人間健康科学科、弊研究室からも出展しました。なかなか盛況でした。 「かたち」からわかる医学 ![]() ![]() 岩佐さんの腹直筋腱画についての論文がJ Anatomyに掲載されました。MRI_DTIを用いて腹直筋腱画形成過程を検討しました。
Iwasa Y, KanahashiT, ImaiH, OtaniH, YamadaS, Takakuwa T. Formation of tendinous intersections in the human fetal rectus abdominis, J Anatomy 2024, in press, DOI: 10.1111/joa.14064 Abstract Previous studies have poorly described the initial development process of the tendinous intersections of the rectus abdominis muscle (RAM). The present study aimed to observe the formation of tendinous intersections in the RAM during the early fetal period using diffusion tensor imaging (DTI). Fifteen human fetal specimens (crown-rump length [CRL]: 39.5–93.7 mm) were selected. Three-dimensional measurements revealed that Zone-4 (i.e., the zone between the pubic symphysis and the caudal base of the umbilical ring in the RAM) had a smaller width and was thicker than Zone-1 and Zone-2 (i.e., the zones between the costal arch and the cranial base of the umbilical ring) and Zone-3 (i.e., the zone at the umbilical ring). Characteristics of tendinous intersections in the RAM during the early fetal period were assessed according to number, size, type, laterality, and sex. The mean number of tendinous intersections on both sides was 3.1 (range: 2.0–4.0), and 21% of specimens had only two tendinous intersections, which was higher than that reported in previous adult studies. The present data suggest that the formation of tendinous intersections was still in progress in specimens with two tendinous intersections in the RAM and that the third tendinous intersection was formed in Zone-2. Ordinal logistic regression via generalized estimating equations revealed that the odds for a higher type of tendinous intersections in Zone-1 and Zone-2 were significantly higher than those in Zone-4 (adjusted odds ratio: 14.85, 8.84). The odds for the presence of incomplete types (tendinous intersections that could not completely transverse the RAM) in Zone-3 were significantly higher than those in Zone-1 (adjusted odds ratio: 7.4). The odds for missing tendinous intersections in Zone-4 were significantly higher than those in Zone-1 (adjusted odds ratio: 20.5). These zonal differences in the formation of tendinous intersections were consistent with those observed in previous adult studies. In this study, DTI detected tendinous intersections in a sample with a CRL of 45.8 mm (approximately 11 weeks of gestation), which is earlier than that in previous histological findings, indicating that the RAM does not have mature tendinous intersections until the 17th week of gestation. In conclusion, DTI could detect the premature differentiation of tendinous intersection formation. Our data may aid in elucidating the developmental processes of tendinous intersections in the RAM. Marieさんの博士学位審査が行われました (7/29; 高井ホール) 堂々とした発表、質疑応答でした。 ![]() ![]() 第64回日本先天異常学会で発表しました(2024.07.26-27, 東京、江戸川区) P-12 ヒト胎児期における大腸の還納、固定時期の解析 P-21 拡散テンソル画像(DTI)を用いたヒト胚子期・胎児期初期における舌筋の形態形成の検討 P-39 拡散テンソル画像を用いた水晶体線維細胞の配向性の検討 |