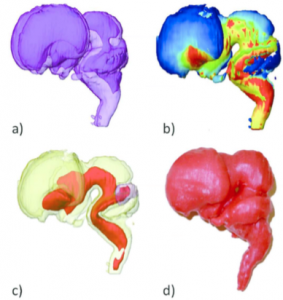
(a) Morphology of the ventricles observed through transparent brain tissue. This processing allowed us to make precise observations of the gross external and internal morphology, including the formation of the ventricular system (Shiraishi et al., 2015a).
(b) Surface color mapping external view of the whole brain. The dynamic changes in thickness on the brain surface were visualized with a color scale (Shiraishi et al., 2015a).
(c) The core region (COR) of the thickening brain wall. The filter module “extracting the core regions,” shrinking from the surface of the brain for constructing COR, enabled visualization of the core regions of the thickening brain wall that resulted from brain differentiation and 3D growth. The brain was relatively uniform in thickness between CS13 and CS16. However, non-uniformity in the thickness of the brain wall was distinct after CS17. The anatomical positions of the COR were mostly consistent with nuclei such as the basal ganglia, thalamus, and pyramidal tract. The COR may be valuable as an anatomical indicator of the development and differentiation of the nuclei and tracts in the brain (Shiraishi et al., 2015a).
(d) Brain Model at CS23. The 3D digital data of MRI (2.35 T) was transformed to .STL data and then subjected to 3D printer (Shiraishi et al., 2013b).
The recent advent of several technologies has enabled us to acquire a much larger field of images with higher resolution. These include MRI and CT, which are acquisition systems; higher specification computers, which can compute a large amount of information accurately in a short time; and higher volume of storage. Using these technologies, datasets from larger samples, which correspond to later part of the first trimester and the second trimester, can be acquired and analyzed. The number of morphological studies on the later part of the first trimester and second trimester are less as compared to those on the first 8 weeks from fertilization (at the end of CS 23)(O’Rahilly and Müller, 1987). Many researchers have been attracted to the dynamic morphogenesis in rather earlier developmental stages. Establishment of CS may contribute in encouraging studies for those periods. In addition, technical limitations may also be the reason to avoid studies on fetuses after CS 23. It is difficult to apply histological analysis for the entire body of the fetus with a size larger than that at CS 23. Studies conducted on post embryonic period are mainly confined to localized histological analysis. From this point of view, 3D datasets of samples in larger samples are worth analyzing as they can reveal the 3D development of the entire body as well as organs.
Our analysis primarily focused on the morphological aspects of digital datasets. Both MRI and PCT contain additional information that reflects the structural component (elements) and structural order (orientation) in addition to the morphology. Previous fetal brain imaging studies using diffusion tensor images have been performed in the second trimester (Huang et al., 2009). The diffusion tensor image method has also been applied to cardiac muscles in mice (Angeli et al., 2014). Such methods maybe are applicable to 3D digital datasets from MRI (7 T), even though the samples have been stored for a long time in formalin. Moreover, PCT with Zeff imaging methods can be used to recognize and differentiate heavy metals such as Fe, Al, Ni, and Cu (Yoneyama et al., 2013). The 3D dynamics of such elements during human embryonic development are not currently known. Hematogenesis of the embryos may be also detectable using Fe as a trace marker. Such information may provide new insight to human development.
When analysis is performed using 3D digitized datasets, the pitfalls and limitations should be known. When a target organ is selected, its internal information and information on its surrounding organs are prone to be lost. Accurate determination of target anatomical landmarks as well as those used for references in digital datasets is very important to increase the accuracy of the analysis and hence that of the conclusion obtained. Careful comparison of histological sections of samples of similar ages and specialized anatomical knowledge are also required.
The 3D information obtained in classical embryology since the late 19th century has been used as the basis of prenatal diagnosis using ultrasound. The use of ultrasound for prenatal diagnostics has rapidly increased in the past 25 years (Blaas 2014). Moreover, 3D sonography performed with high-frequency transvaginal transducers has expanded as 3D sonoembryology. Normal developmental data during the embryonic stages, however, is still insufficient for guiding such clinical evaluations. The 3D analysis in our study may serve to provide accurate morphologic data as well as the dynamics of embryonic structures related to developmental stages required for insights into the dynamic and complex processes occurring during organogenesis.
Literature cited
Angeli S, Befera N, Peyrat JM, Calabrese E, Johnson GA, Constantinides CA. 2014. High-resolution cardiovascular magnetic resonance diffusion tensor map from ex-vivo C57BL/6 murine hearts. J Cardiovasc Magn Reson 16:77.
Blaas HG. 2014. Detection of structural abnormalities in the first trimester using ultrasound. Best Pract Res Clin Obstet Gynaecol 28:341-353.
Desmond ME, O’Rahilly R. 1981. The growth of the human brain during the embryonic period proper. 1. Linear axes. Anat Embryol (Berl) 162:137-151.
Endo A, Ueno S, Yamada S, Uwabe C, Takakuwa T. 2015. Morphogenesis of the spleen during the human embryonic period. Anat Rec 298:820-826.
Gasser RF, Cork RJ, Stillwell BJ, McWilliams DT. 2014. Rebirth of human embryology. Dev Dyn 243:621-628.
Gasser RT. 2006. Evidence that some events of mammalian embryogenesis can result from differential growth, making migration unnecessary. Anat Rec B 289B:53-63.
Hamabe Y, Hirose A, Yamada S, Uwabe C, Okada T, Togashi K, Kose K, Takakuwa T. 2013. Morphology and morphometry of fetal Liver at 16-26 weeks of gestation by magnetic resonance imaging- comparison with embryonic liver at Carnegie Stage 23. Hepatol Res 43:639-647.
Hirose A, Nakashima T, Shiraki N, Yamada S, Uwabe C, Kose K, Takakuwa T. 2015. 3D models related to the publication: Morphogenesis of the liver during the human embryonic period. MorphoMuseuM 1(4):e1.
Hirose A, Nakashima T, Yamada S, Uwabe C, Kose K, Takakuwa T. 2012. Embryonic liver morphology and morphometry by magnetic resonance microscopic imaging. Anat Rec (Hoboken) 295:51-59.
Huang H, Xue R, Zhang J, Ren T, Richards LJ, Yarowsky P, Miller MI, Mori S, 2009. Anatomical characterization of human fetal brain development with diffusion tensor magnetic resonance imaging. J Neurosci 29:4263-4273.
Kagurasho M, Yamada S, Uwabe C, Kose K, Takakuwa T. 2012. Movement of the external ear in human embryo. Head Face Med 8:2.
Kaigai N, Nako A, Yamada S, Uwabe C, Kose K, Takakuwa T. 2014. Morphogenesis and three-dimensional movement of the stomach during the human embryonic period. Anat Rec (Hoboken) 297:791-797.
Kanahashi T, Yamada S, Tanaka M, Hirose A, Uwabe C, Kose K, Yoneyama A, Takeda T, Takakuwa T. 2016. A novel strategy to reveal the latent abnormalities in human embryonic stages from a large embryo collection. Anat Rec 299:8-24.
Kerwin J, Yang Y, Merchan P, Sarma S, Thompson J, Wang X, Sandoval J, Puelles L, Baldock R, Lindsay S. 2010. The HuDSEN Atlas: a three-dimensional (3D) spatial framework for studying gene expression in the developing human brain. J Anat 217:289-299.
Kishimoto H, Yamada S, Kanahashi T, Yoneyama A, Imai H, Matsuda T, Takeda T, Kawai K. 2016. Three-dimensional observation of palatal muscles in the human embryo and fetus: development of levator veli palatini and clinical importance of the lesser palatine nerve. Dev Dynam 245:123-131.
Kobayashi A, Ishizu K, Yamada S, Uwabe C, Kose K, Takakuwa T. 2016. Morphometric human embryonic brain features according to developmental stage. Prenat Diagn 36:338-345.
Komori M, Miura T, Shiota K, Minato K, Takahashi T. 1995. Virtual embryology: a 3D library reconstructed from human embryo sections and animation of development process. MEDINFO 8 Pt 2:1229-1230.
Levitan ML, Desmond ME. 2009. Expansion of the human embryonic brain during rapid growth: area analysis. Anat Rec 292:472-480.
Matsuda Y, Ono S, Otake Y, Handa S, Kose K, Haishi T, Yamada S, Uwabe C, Shiota K. 2007. Imaging of a large collection of human embryo using a super-parallel MR microscope. Magn Reson Med Sci 6:139-146.
Matsuda Y, Utsuzawa S, Kurimoto T, Haishi T, Yamazaki Y, Kose K, Anno I, Marutani M. 2003. Super-parallel MR microscope. Magn Reson Med 50:183-189.
Mehemed TM, Fushimi Y, Okada T, Kanagaki M, Okada T, Yamamoto A, Takakuwa T, Yamada S, Togashi K. 2016. Magnetic resonance imaging of pituitary gland and postsphenoid ossification in fetal specimens. Am J Neuroradiology 37: 1523-1527.
Morgan LM. 2004. A social biography of Carnegie embryo no. 836. Anat Rec B New Anat 276:3-7.
Nakashima T, Hirose A, Yamada S, Uwabe C, Kose K, Takakuwa T. 2012. Morphometric analysis of the brain vesicles during the human embryonic period by magnetic resonance microscopic imaging. Congenit Anom (Kyoto) 52:55-58.
Nako A, Kaigai N, Shiraki N, Yamada S, Uwabe C, Kose K, Takakuwa T. 2016. 3D models related to the publication: Morphogenesis of the stomach during the human embryonic period. MorphoMuseuM 1(4):e3.
Nishimura H, Takano K, Tanimura T, Yasuda M. 1968. Normal and abnormal development of human embryos: first report of the analysis of 1,213 intact embryos. Teratology 1:281-290.
O’Rahilly R, Müller F. 2010. Developmental stages in human embryos: revised and new measurements. Cells Tiss Org 292:73-84.
O’Rahilly R, Müller F. 1987. Developmental stages in human embryos: including a revision of Streeter’s Horizons and a survey of the Carnegie Collection. Washington, D.C.: Carnegie Institution of Washington. p 239-251.
Osaka M, Ishikawa A, Yamada S, Uwabe C, Imai H, Matsuda T, Yoneyama A, Takeda T, Takakuwa T. 2017. Positional changes of the ocular organs during craniofacial development. Anat Rec, 300(12), 2107–2114, 2017 DOI: 10.1002/ar.23588
Ozeki-Sato M, Yamada S, Uwabe C, Ishizu K, Takakuwa T. 2016a. Correlation of external ear auricle formation with staging of human embryos. Congenit Anom (Kyoto) 56:86-90.
Ozeki-Sato M, Ishikawa A, Yamada S, Uwabe C, Takakuwa T. 2016b. Morphogenesis of the Middle Ear Ossicles and Spatial Relationships with the External and Inner Ears during the Embryonic Period. Anat Rec 299: 1325-1337.
Shimizu A, Hasegawa I, Kobayashi N, Shono H, Nawano S, Sato I, Komagata H, Ishikawa M, Shinoda K. 2016. Fundamental technologies for integration of multiscale spatiotemporal morphology in multidisciplinary computational anatomy. Progress Overview FY2015. Proceedings of the 2nd International Symposium on Multidisciplinary Computational Anatomy, 17-22.
Shiraishi N, Katayama A, Nakashima T, Yamada S, Uwabe C, Kose K, Takakuwa T. 2015a. Morphology and morphometry of the human embryonic brain: A three-dimensional analysis. NeuroImage 115:96-103.
Shiraishi N, Katayama A, Nakashima T, Shiraki N, Yamada S, Uwabe C, Kose K, Takakuwa T. 2015b. 3D model related to the publication: Morphology of the human embryonic brain and ventricles. MorphoMuseuM 1(3):e3.
Shiraishi N, Katayama A, Nakashima T, Yamada S, Uwabe C, Kose K, Takakuwa T. 2015c. Three-dimensional morphology of the human embryonic brain. Data in Brief 4:116-118.
Shiraishi N, Nakashima T, Yamada S, Uwabe C, Kose K, Takakuwa T. 2013a. Morphogenesis of lateral choroid plexus during human embryonic period. Anat Rec (Hoboken) 296:692-700.
Shiraishi N, Yamada S, Takakuwa T. 2013b. Three-dimensional models once again – for research and teaching of early human development. Congenit Anom (Kyoto) 53:58-59.
Shiota K, Yamada S, Nakatsu-Komatsu T, Uwabe C, Kose K, Matsuda Y, Haishi T, Mizuta S, Matsuda T. 2007. Visualization of human prenatal development by magnetic resonance imaging (MRI). Am J Med Genet A 143A:3121-3126.
Shiota K. 1991. Development and intrauterine fate of normal and abnormal human conceptuses. Congenit Anom 31:67-80.
Smith BR. 1999. Visualizing human embryos. Scientific Am 280, 76-81.
Takakuwa T, Koike T, Muranaka T, Yamada S, Uwabe C. 2016a. Formation of the circle of Willis during human embryonic development. Congenit Anom (Kyoto) 56: 233-236.
Takakuwa T, Muranaka T, Yamada S. 2016b. Three-dimensional Analysis of the Bronchial Branching in Human Embryonic Stages. Progress Overview FY2015. Proceedings of the 2nd International Symposium on Multidisciplinary Computational Anatomy. 43-46.
Taketani K, Yamada S, Uwabe C, Okada T, Togash K, Takakuwa T. 2015. Morphological features and length measurements of fetal lateral ventricles at 16–25 weeks of gestation by magnetic resonance imaging. Congenit Anom (Kyoto) 55:99-102.
Toyoda S, Shiraki N, Yamada S, Uwabe C, Imai H, Matsuda T, Yoneyama A, Takeda T, Takakuwa T. 2015a. Morphogenesis of the human inner ear membranous labyrinth. MorphoMuseuM 1(3):e6.
Toyoda S, Shiraki N, Yamada S, Uwabe C, Imai H, Matsuda T, Yoneyama A, Takeda T, Takakuwa T. 2015b. Morphogenesis of the inner ear at different stages of normal human development. Anat Rec (Hoboken) 298:2081-2090.
Ueda Y, Yamada S, Uwabe C, Kose K, Takakuwa T. 2016. Intestinal rotation and physiological umbilical herniation during the embryonic period. Anat Rec (Hoboken) 299:197-206.
Ueno S, Yamada S, Uwabe C, Männer J, Shiraki N, Takakuwa T. 2016. The digestive tract and derived primordia differentiate by following a precise timeline in human embryos between Carnegie stages 11 and 13. Anat Rec (Hoboken) 299:439-449.
Yamada S, Samtani RR, Lee ES, Lockett E, Uwabe C, Shiota K, Anderson SA, Lo CW. 2010. Developmental atlas of the early first trimester human embryo. Dev Dyn 239:1585-1595.
Yoneyama A, Hyodo K, Takeda T. 2013. Feasibility test of Zeff imaging using x-ray interferometry. Appl Phys Lett 103:108.
Yoneyama A, Amino N, Mori M, Kudoh M, Takeda T, Hyodo K, Hirai Y. 2006. Non-invasive and time-resolved observation of tumors implanted in living mice by using phase-contrast X-ray computed tomography. Jpn J Appl Phys 45:1864.
Yoneyama A, Takeda T, Tsuchiya Y, Wu J, Lwin TT, Koizumi A, Hyodo K, Itai Y. 2004. A phase-contrast X-ray imaging system-with a 60 x 30 mm field of view based on a skew-symmetric two-crystal X-ray interferometer. Nucl Instrum Methods Phys Res A523:217-222.